Orbital engineering of strongly correlated oxides
Orbitals are visualized as the shape or form that an electron cloud
takes in a particular quantum state. In transition metal oxides, the
nature of the anisotropic d orbitals determines several crucial
parameters such as hopping strength, exchange interaction, correlation,
covalency, superconducting pairing symmetry etc. Thus, the study of
orbital physics is crucial for understanding the mechanism behind
multitude of phenomena such as, metal-insulator transition,
superconductivity, magnetism, colossal magnetoresistance etc, hosted by
transition metal oxides.
In recent times, the advancement of thin
film growth technique has enabled the manipulation of these orbitals
through heterostructuring and has opened a new route to create and
control different phases of matter. Our goal is not only to reveal the
orbital physics of various strongly correlated systems but also to
devise novel phases in artificial quantum materials through orbital
engineering.
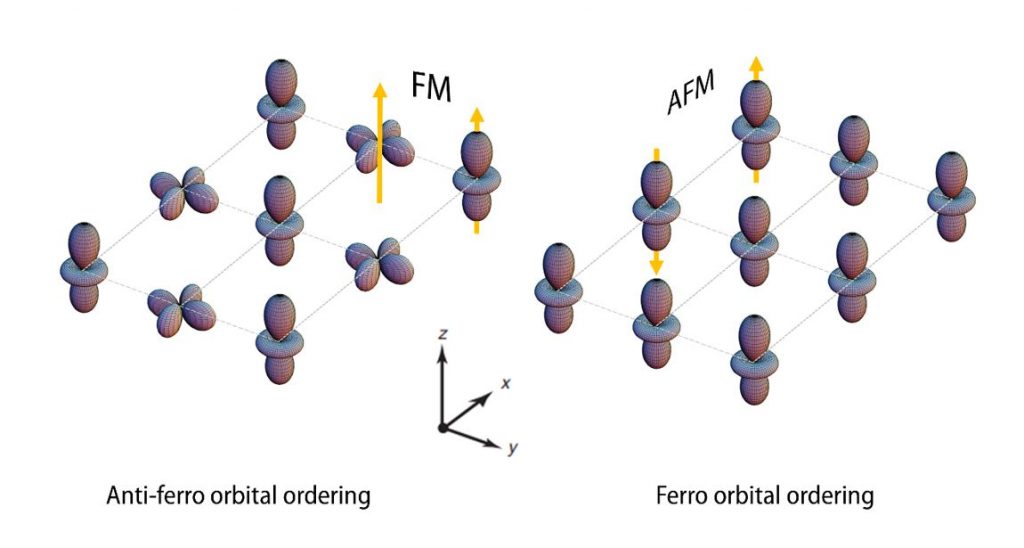
Review paper on this topic: D. I. Khomskii, ECS J. Solid State Sci. Technol. 11, 054004 (2022).
Our recent work on this theme: Mandal#, Patel# et al. Phys. Rev. B (Letters) 103, L060504 (2021); Mandal et al., Phys. Rev. B 108, 045145 (2023)
Two dimensional electron gas
Electrons confined in two dimension exhibit a plethora of fascinating electronic and magnetic phases and forms the basis of current electronic industry. An interesting subclass of such a large family is the emergent two-dimensional electron gas (2-DEG) confined at the interface between two complex oxides. The shorter confinement length of the carriers along with broken inversion symmetry in such artificial structures leads to several tunable novel quantum phases, which are unattainable in traditional semiconductor based 2-DEGs. This makes complex oxide based 2-DEGs an attractive platform for quantum device application.
Our group work on variety of phenomena such as superconductivity, non-trivial spin structures and domain boundary engineering, which have marked technological applications and would be monumental in revolutionizing the field of oxide electronics.
Review paper on this topic: Stemmer et al. Annual Review of Materials Research, 44, 151 (2014).
Our recent work on this theme: Ojha, et al. Adv. Quant. Tech. 3, 2000021 (2020); Ojha#, Gogoi# et al. Phys. Rev. B, 103, 085120 (2021); Ojha, et al. Phys. Rev. Appl. 15, 054008(2021); Mandal, et al. J. Phys. Chem. C, 125, 12968 (2021); Ojha#, P. Mandal# et al. Communication Physics (2023).
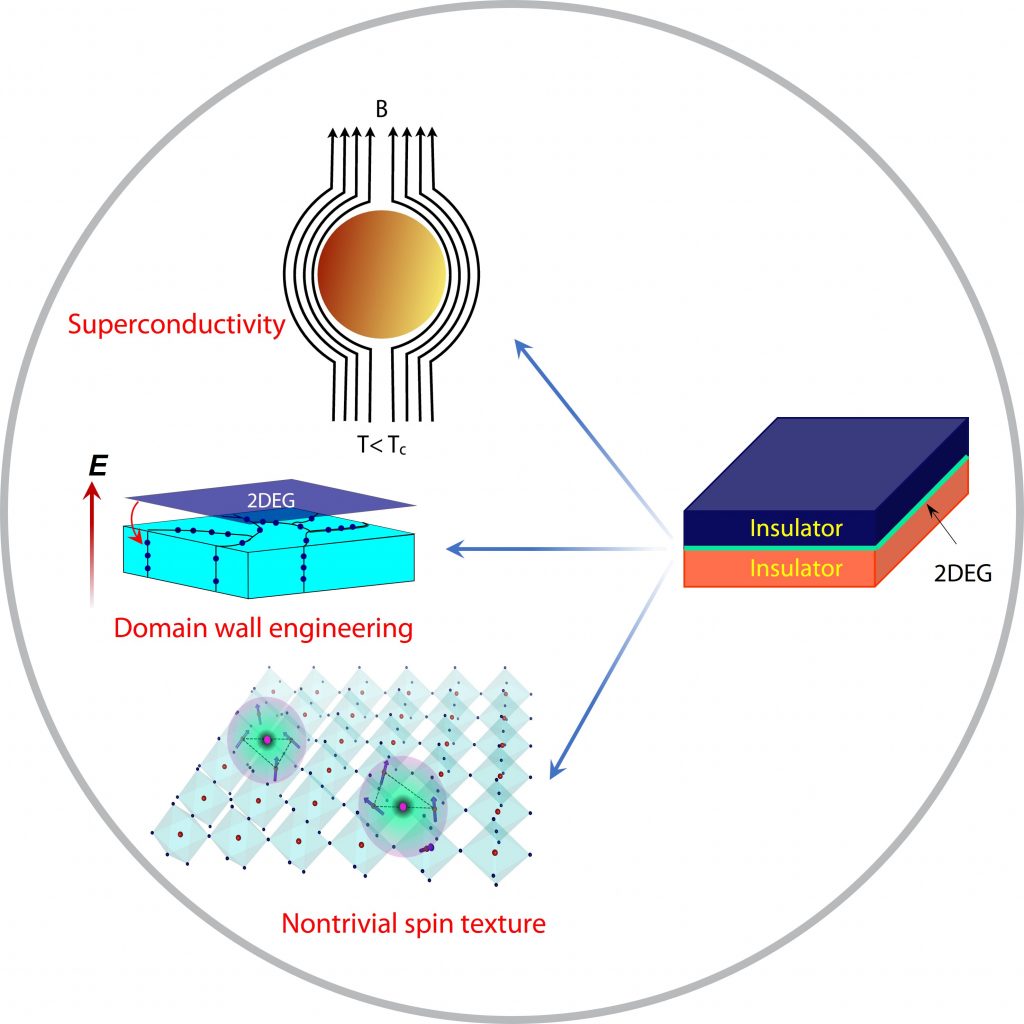
High entropy oxide
The recent development of machine learning and availability of huge materials database enable us to predict new materials with desired properties that are not realised yet. One such promising class of material is high entropy oxides (HEOs). HEOs are a class of materials containing equimolar or nearly equimolar portions of five or more elements occupying the same crystallographic site. These HEO materials have gained significant interest in recent years as they offer opportunity to achieve tunable properties in unexplored parts of the complex phase diagram. Also, they are potential platforms for applications such as energy storage devices, fuel cells, solar cells, super capacitors, dielectric applications, etc.
However, the thin-film growth of such multi-component systems in a single crystalline form is extremely challenging due to the difficulty in stabilizing in a single phase. We are working on finding such HEO compounds and studying the effect of disorder on the electronic and magnetic properties in the thin film geometry.
Review paper on this topic: Musico et al., APL Materials 8, 040912 (2020).
Our recent work on this theme: R. K. Patel et al. Applied Physics Letters 116, 071601 (2020); Appl. Phys. Rev. (2023)
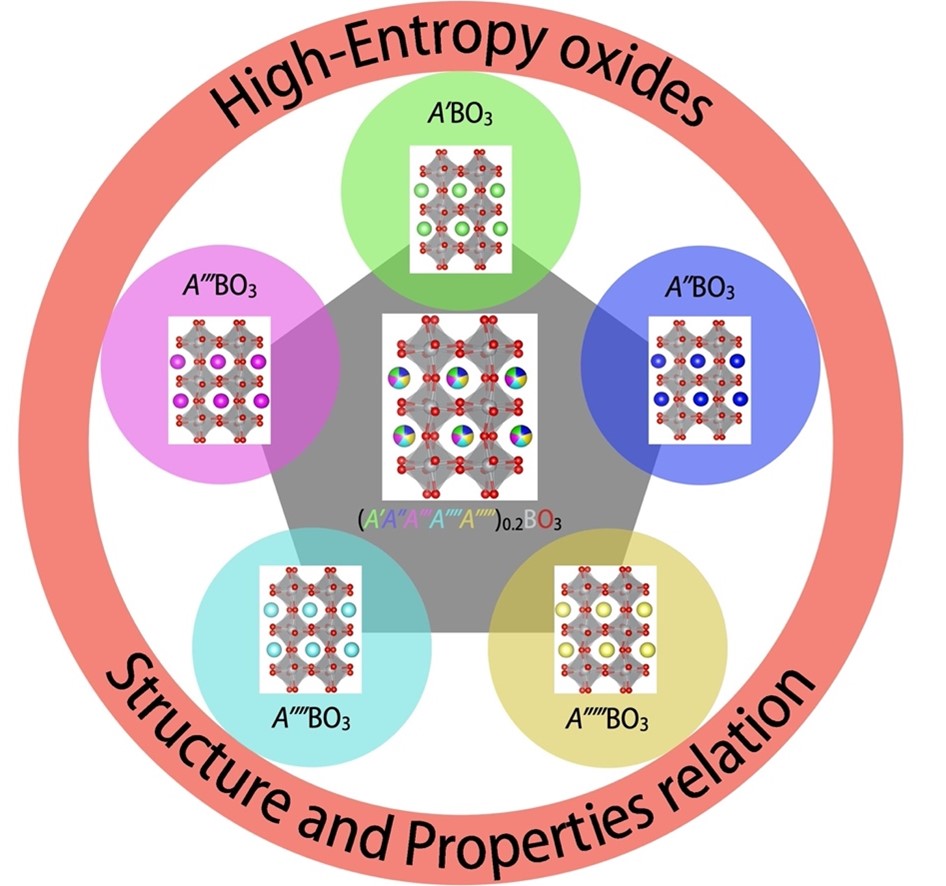