Quantum transport in Graphene
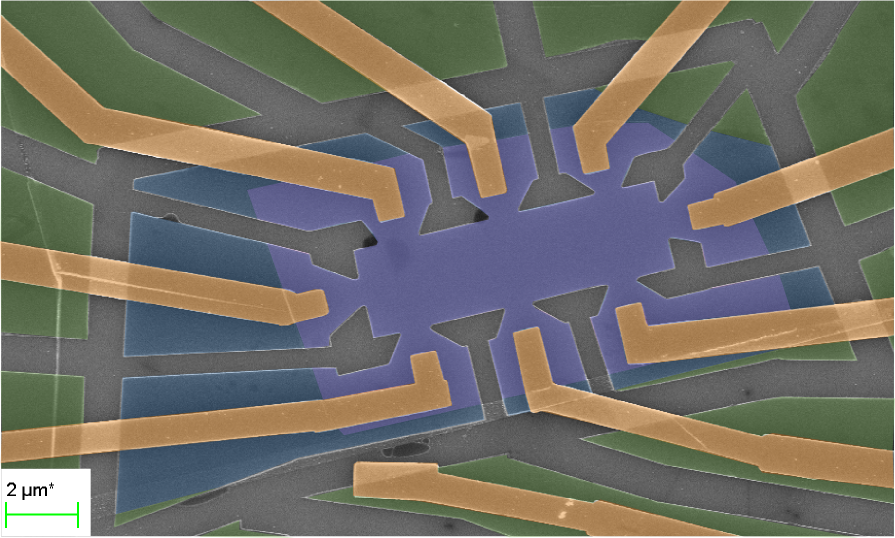
Universality of quantum phase transitions in the integer and fractional quantum Hall regimes Nature Communications (2024)
Fractional quantum Hall (FQH) phases emerge due to strong electronic interactions and are characterized by anyonic quasiparticles, each distinguished by unique topological parameters, fractional charge, and statistics. In contrast, the integer quantum Hall (IQH) effects can be understood from the band topology of non-interacting electrons. We report a surprising super-universality of the critical behavior across all FQH and IQH transitions. Contrary to the anticipated state-dependent critical exponents, our findings reveal the same critical scaling exponent κ = 0.41 ± 0.02 and localization length exponent γ = 2.4 ± 0.2 for fractional and integer quantum Hall transitions. From these, we extract the value of the dynamical exponent z ≈ 1. We have achieved this in ultra-high mobility trilayer graphene devices with a metallic screening layer close to the conduction channels. The observation of these global critical exponents across various quantum Hall phase transitions was masked in previous studies by significant sample-to-sample variation in the measured values of κ in conventional semiconductor heterostructures, where long-range correlated disorder dominates. We show that the robust scaling exponents are valid in the limit of short-range disorder correlations.
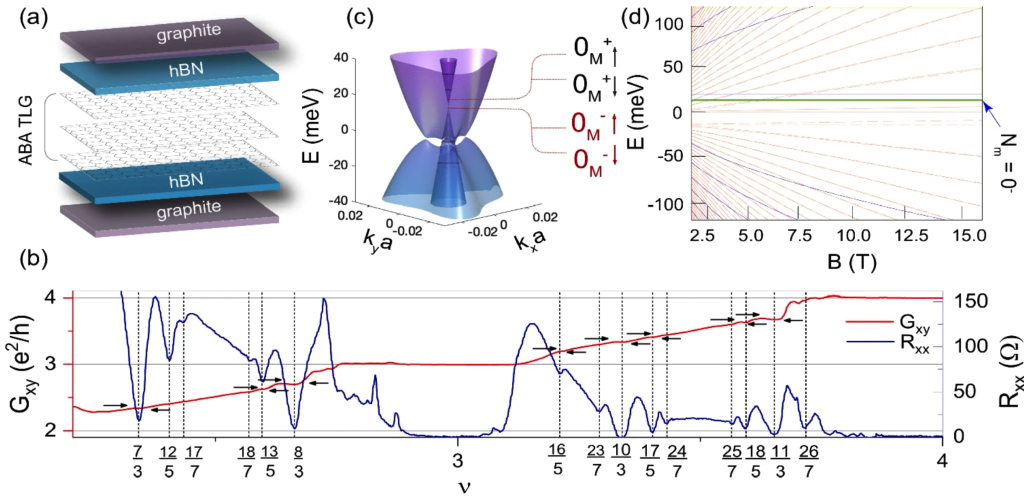
Observation of the Time-Reversal Symmetric Hall Effect in Graphene–WSe2 Heterostructures at Room Temperature (pdf) Nano Letters (2023)
In this Letter, we provide experimental evidence of the time-reversal symmetric Hall effect in a mesoscopic system, namely, high-mobility graphene–WSe2 heterostructures. This linear, dissipative Hall effect, whose sign depends on the sign of the charge carriers, persists up to room temperature. The magnitude and the sign of the Hall signal can be tuned using an external perpendicular electric field. Our joint experimental and theoretical study establishes that the strain induced by lattice mismatch, or alignment angle inhomogeneity, produces anisotropic bands in graphene while simultaneously breaking the inversion symmetry. The band anisotropy and reduced spatial symmetry lead to the appearance of a time-reversal symmetric Hall effect. Our study establishes graphene–transition metal dichalcogenide-based heterostructures as an excellent platform for studying the effects of broken symmetry on the physical properties of band-engineered two-dimensional systems.
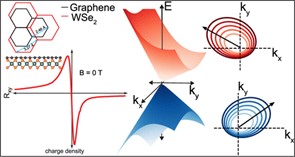
Experimental observation of spin-split energy dispersion in high-mobility single-layer graphene/WSe2 heterostructures (pdf) npj 2D Materials and Applications (2022). (Post on the article)
Proximity-induced spin-orbit coupling in graphene has led to the observation of intriguing phenomena like time-reversal invariant Z2 topological phase and spin-orbital filtering effects. An understanding of the effect of spin-orbit coupling on the band structure of graphene is essential if these exciting observations are to be transformed into real-world applications. In this research article, we report the experimental determination of the band structure of single-layer graphene (SLG) in the presence of strong proximity-induced spin-orbit coupling. We achieve this in high-mobility hexagonal boron nitride (hBN)-encapsulated SLG/WSe2 heterostructures through measurements of quantum oscillations. We observe clear spin-splitting of the graphene bands and a substantial increase in the Fermi velocity. Using a theoretical model with realistic parameters to fit our experimental data, we uncover evidence of a band gap opening and band inversion in the SLG. Further, we establish that the deviation of the low-energy band structure from pristine SLG is determined primarily by the valley-Zeeman SOC and Rashba SOC, with the Kane–Mele SOC being inconsequential. Despite robust theoretical predictions and observations of band-splitting, a quantitative measure of the spin-splitting of the valence and the conduction bands and the consequent low-energy dispersion relation in SLG was missing—our combined experimental and theoretical study fills this lacuna.
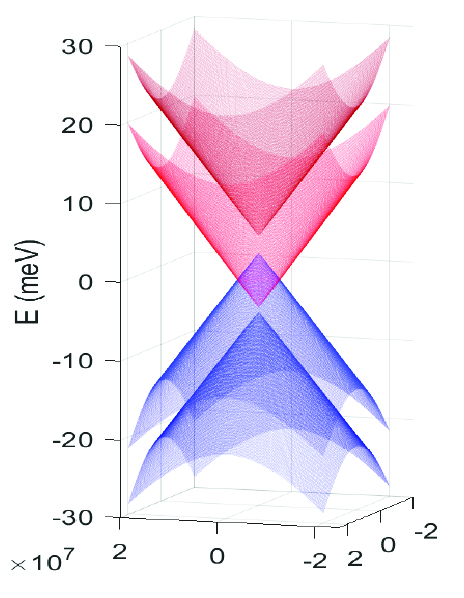
Time-Reversal Invariant Helical Edge-Modes in Bilayer Graphene/WSe2 Heterostructure ACS Nano 15 916, (2021)
The Quantum Spin Hall (QSH) insulator is a zero-magnetic field version of the Quantum Hall (QH) effect. It is characterized by helical edge modes, as opposed to chiral edge modes in the case of QH. Kane and Mele, in a seminal paper in 2005, had predicted that in single-layer graphene with strong spin-orbit interaction (SOI) one should be able to observe QSH insulator phase. This prediction (in conjunction with the work of Haldane) was, in some sense, the genesis of the field od of topological insulators. Although QSH state was experimentally seen in several semiconducting materials, observation of intrinsic, time-reversal invariant QSH in graphene remained elusive due to the minimal strength of SOI in graphene.
We recently observed QSH in bilayer graphene (BLG). The device consisted of a van der Waals heterostructure of BLG and single-layer WSe2 encapsulated between layers of hexagonal BN. The presence of WSe2 induced strong SOI in the BLG as seen from several indicators – SdH oscillations, weak anti-localization correction to low-field magnetoconductivity, asymmetry in the measured band-gap, and large SPin Hall signal. We measured exactly quantized conductance in several different measurement configurations, the results in each case matching with that expected for QSH state in BLG.

Electric-field-tunable valley Zeeman effect in bilayer graphene heterostructures: Realization of the spin-orbit valve effect Phys. Rev. Letts. 126 096801 (2021).
We report the discovery of electric-field-induced transition from a topologically trivial to a topologically nontrivial band structure in an atomically sharp heterostructure of bilayer graphene (BLG) and single-layer WSe2 per the theoretical predictions of Gmitra and Fabian [Phys. Rev. Lett. 119, 146401 (2017)]. Through detailed studies of the quantum correction to the conductance in the BLG, we establish that the bandstructure evolution arises from an interplay between proximity-induced strong spin-orbit interaction (SOI) and the layer polarizability in BLG. The low-energy carriers in the BLG experience an effective valley Zeeman SOI that is completely gate-tunable to the extent that it can be switched on or off by applying a transverse displacement field or can be controllably transferred between the valence and the conduction band. We demonstrate that this results in the evolution from weak localization to weak antilocalization at a constant electronic density as the net displacement field is tuned from a positive to a negative value with a concomitant SOI-induced splitting of the low-energy bands of the BLG near the K(K’) valley, which is a
unique signature of the theoretically predicted spin-orbit valve effect. Our analysis shows that quantum correction to the Drude conductance in Dirac materials with strong induced SOI can only be explained satisfactorily by a theory that accounts for the SOI-induced spin splitting of the BLG low-energy bands. Our results demonstrate the potential for achieving highly tunable devices based on the valley Zeeman effect in dual-gated two-dimensional materials.
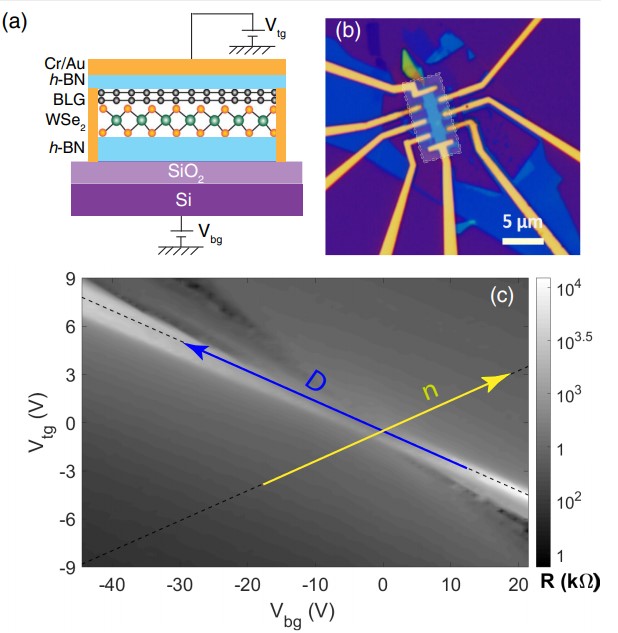
Fractional Quantum Hall Effect
To date, the fractional quantum Hall effect (FQHE) had almost exclusively been studied on high-mobility two-dimensional electronic gas (2DEG) based on GaAs/AlGaAs heterostructures. High-quality materials of such kind are typically made using epitaxial growth methods like Molecular Beam Epitaxy (MBE). These methods, while producing very high-quality devices cannot be used for high volume CMOS production as they have a relatively slow production rate and are very expensive. This and the requirement of ultra-low temperatures for the observation of FQHE in such materials have impeded any attempts to translate their interesting properties into practical device applications. The recent observation of FQHE in graphene and in two-dimensional electron gases in oxide-oxide interfaces has opened up new possibilities in this field. Quantum Hall Effect in graphene has unique characteristics due to the fourfold spin and valley (pseudo-spin) degeneracy of Landau levels and the existence of a non-trivial Berry phase associated with the pseudo-spin of Dirac quasiparticles. For example, due to the SU(4) symmetry of graphene, novel FQHE states are predicted that have no analog in GaAs/AlGaAs systems. Despite the apparent similarity of the observed 1/3 state in graphene with that seen for the non-relativistic electrons in GaAs/AlGaAs 2DEG, it is not obvious that the correlated states in both cases are the same. In fact, several different competing scenarios have been proposed for FQHE in graphene – from breaking of the SU(4) symmetry to the formation of a Composite Fermion kind of fluid. It would thus be interesting to see how much of our understanding of the quantum hall effect (and of mesoscopic transport in general) can be carried over to the massless Dirac particles in graphene. Some of our related work in this field are summarized below:
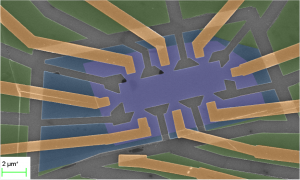